Application note AN22_02
Isotopx Ltd, Dalton House, Dalton Way, Middlewich, Cheshire, CW10 0HU, UK.
How can SIRIX be used to improve CO2 measurement?
Introduction
The objective of CO2 isotopologue studies is to accurately and precisely measure small differences (Δ 47) in the abundance of m/z 47 (13C18O16O) relative to m/z 44 12C16O16O. Small changes in Δ47, are related to changes in temperature of formation of carbonate rocks1 . The relationship between temperature and Δ47 can be defined by the equation1:
Δ47 = 0.0592 x 106 x T-2 -0.02
Where T is the Temperature in Kelvin and Δ47 is in per mil (1/1000). This is depicted in Figure 1 below.
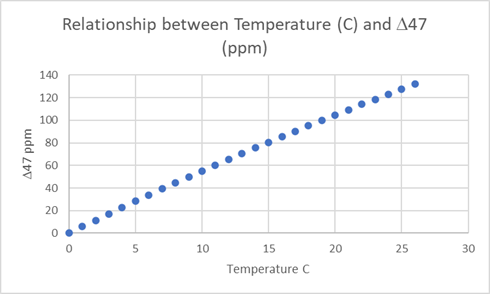
The isotope ratio 47/44 is approximately 4.5e-5. The mass spectrometric challenge to achieve these precisions include:
- A highly stable ion source
- High sensitivity from the ion source
- High resolution ion optics
- Flexible collector configuration to allow continuous baseline measurement
- No baseline aberrations from ion collisions with the flight tube
- Low noise, high dynamic range Faraday amplifier
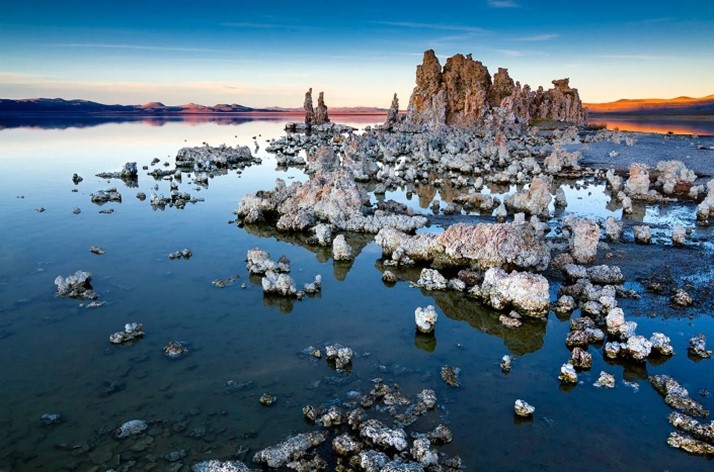
SIRIX large radius stable isotope ratio mass spectrometer
The SIRIX mass spectrometer design is essentially a Phoenix thermal ionization mass spectrometer, with the Thermal Ionisation source removed and replaced with a high efficiency electron bombardment gas source.
The SIRIX ion source is constructed of high purity stainless steel. It is pumped with a 300 l/s turbomolecular pump backed with an oil-free scroll pump. Ions are accelerated through a potential of 10 KV, through an exit slit of 0.3 mm width. Ions are focused in the X-Y-Z direction.
The large electromagnet produces a mass dispersion of 610 mm, this was designed for TIMS applications, but is well suited for CO2 isotopologue studies as the isotope peaks are well separated at the collector, thereby reducing peak tailing. The base mass resolution of the SIRIX is 600 (10% valley) which is higher than most other gas source instruments. The high resolution ensures that hydrocarbons cannot interfere with peak centres.
An example of the peak shape and collector coincidence is shown in Figure 2. All collectors have 1mm entrance slits resulting in the same peak widths across the collector. Flat topped peaks are generated because the ion beam width is 0.3 mm.
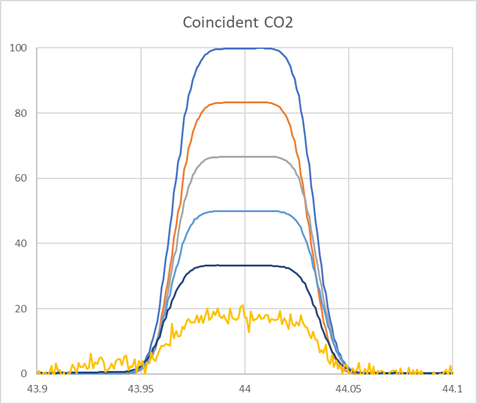
Flexible ion collection
A feature of the SIRIX is that all nine Faraday collectors can be moved independently. There is no fixed collector spacing. Thus no compromise in peak positioning and each isotope system is ensured of perfect peak coincidence.
In the context of CO2 isotopologue measurements, not only can the isotopologues of CO2 be measured in STATIC multicollection mode, but at the same time the half-mass baselines can be measured between the minor isotope peaks. This is shown in Table 1 below.
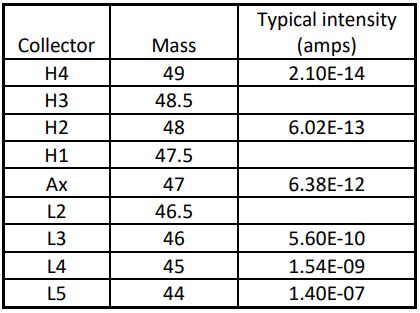
Baseline (peak tailing) measurement
The importance of accurate baseline measurement is demonstrated in Figure 3, which shows the high mass peak tailing from the m/z 44 peak beneath the minor isotopes 47, 48 and 49. The 44 signal is 1.4e-7 amps. The 47 peak is off-scale at 6.3e-12 amps. The peak tail at mass 47 is 5e-15 amps, so without a tail correction the contribution of the peak tail under mass 47 is approximately 0.1%.
At mass 48 the uncorrected peak tail is approximately 1% of the 48 peak. There is no evidence of any other baseline artefacts, for example from collisions with the flight tube. These reflections would produce larger errors in isotope ratios with increased ion intensity and would prevent an improvement in precision with increasing ion intensity. We believe the lack of reflections is due to internal baffling and the wide flight tube.
The amount of peak tail can be seen relative to the m/z 49 peak in Figure 2. The 49 peak is 0.14 ppm of mass 44. Therefore, at mass 47 it is 0.07 ppm relative to 44, at mass 48 it is 0.03 ppm and <0.01 ppm at mass 49. All the peaks are clearly resolved from the peak tail.
Table 1 shows that for this application the peak tail at mass 47 can be exactly quantified by measuring m/z 46.5 and 47.5. By taking the average of the two intensities a precise baseline correction can be made. For mass 48 the baseline is measured at 47.5 and 48.5. For 49 a baseline at 48.5 is used. Baseline subtraction can be made for each integration. This is important because during analysis the ion signal is constantly reducing, and baselines taken at the start of the measurement are not applicable during the measurement.
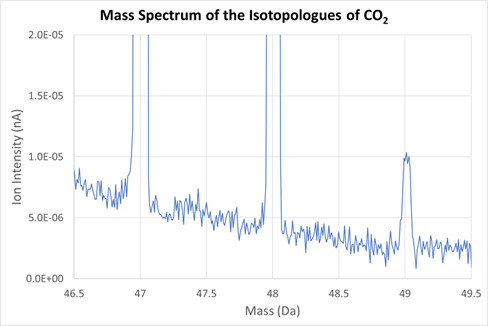
ATONA amplifier technology
The ATONATM amplifier technology has been described elsewhere. In the example of isotopologue measurements, the ATONA is particularly well suited. The extremely large ion intensities of the major isotopes (Table 1) can be measured using the same detectors as the minor isotopes without range changing or calibration. Features of the ATONA include:
- Extremely rapid response; maximum signal to baseline in less than 300 ms
- Dynamic range of ten orders of magnitude
- Gain stability of <2 ppm 2RSD over 24 hours
- Noise stability of ~1e-18 amps over an hour.
CO2 measurements
Figure 4 Shows typical ion beam behavior over the course of 100 minutes. Each data point is a 1 second integration. A total of 6000 integrations were made, in 10 blocks of 600. The ion beam was peak centered at the start of each block. The ion signal of the 44 CO2 starts at just over 1000 volts (relative to a 1e11 ohm resistor) or 1e-8 amps. It decays slowly over the period by about 10%. No ion focusing was done during the acquisition. The mass 47 isotope was 0.4V, or 4e-12 amps. This is too high for a 1e13 ohm resistor but could be measured with a 1e12 ohm resistor.
The 45/44 isotope ratio shows some fine structure during the acquisition, and this is also seen within the 47/44 data set. We believe this is due to mass fractionation effects within the ion source.



The final results are shown in Table 2 below.
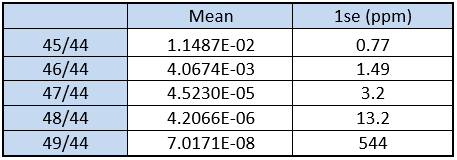
Results
The results can be further broken down into individual 10 minute, 600 cycle integrations. This is shown in Table 3. Individual data blocks produce 47/44 data of sub-10 ppm 1rse, while the major isotopes have precisions of less than 2 ppm 1rse. The reproducibility expressed as 1RSD is not as good as this. For example, the reproducibility of 45/44 is 54 ppm, for 46/44 it is 107 ppm and for 47/44 it is 168 ppm.
The increase is entirely related to the mass difference between the isotopes and not the absolute abundance of the isotope. Despite the 47 isotope being two orders of magnitude smaller than the 46 isotope, the reproducibility of the data is controlled by mass fractionation. This is further illustrated in Figure 5, where a strong correlation is shown between 47/44 and 45/44 and follows a trajectory of mass fractionation with the loss of the lighter 44 isotope. The corresponding data summary is shown below.
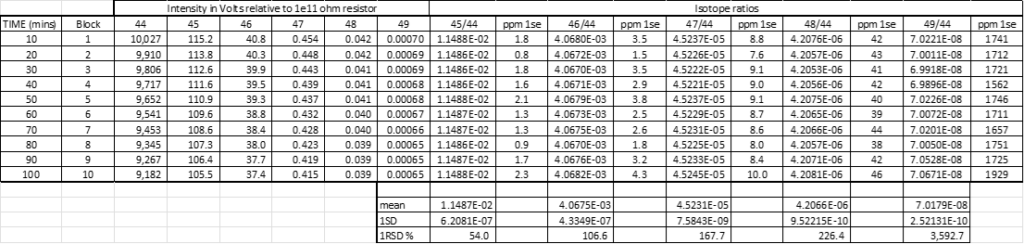
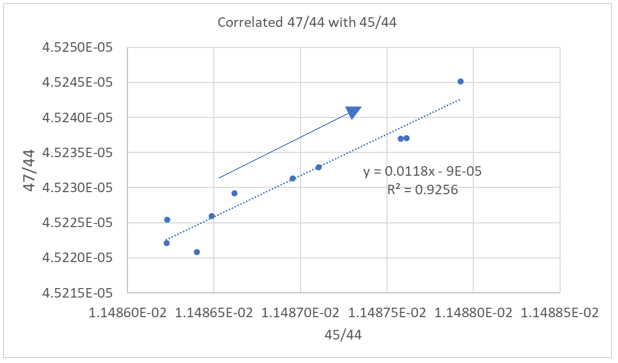
Summary
SIRIX can measure all the isotopes of CO2 with high precision and accuracy. Large ion signals in excess of 1000 volts relative to a 1e11 ohm resistor (>1e-7 amps) can be measured with ATONA. Peak tailing from mass 44 is not a significant source of error. 47/44 precisions of ~3 ppm 1rse can be generated. Higher precision is limited to accurate correction of mass fractionation produced in the ion source.
Reference
1. P. Ghosh, J. Adkins, H. Affek, B. Balta, W. Guo, E. Schauble, E.D.Schrag. 13C-18O bonds in carbonate minerals: A new kind of palaeothermometer. Geochemica et Cosmochimica Acta 2006. 70. 1439.
Authors and Contact Information
David Rousell, Zenon Palacz
Download Technical Note
Download the complete Technical Note:
Measurement of clumped CO2 isotopologues using SIRIX stable isotope ratio mass spectrometer