Zenon Palacz, Shaun Yardley, Matt Hockley, Steve Guilfoyle
What is this study about?
The performance characteristics of an Isotopx Phoenix equipped with 10 conversion dynode ion counters with newly developed MICX electronics is evaluated. This design allows the simultaneous measurement of actinides at unit mass spacing.
The ion counters are independently movable allowing the study of a wide range of isotope systems. This instrument also has a Zeptona Faraday detector. This allows calibration of ion counter gain relative to a Faraday collector at count rates of <~10,000cps, far lower than is possible for resistor-based Faraday collectors.
Background
When measuring very small ion signals (<10,000cps), in sub-picogram samples of actinides, ion counting detectors are necessary due to their low noise compared to that of a Faraday detector. Ion counters, however, still have a non-zero noise associated with them, known as the dark noise, which is typically several counts per minute (CPM). Additionally, there are sources of measurement error present that are not of concern with Faraday detectors, such as deadtime/linearity, peak flatness, and gain stability. Isotopx developments in the MICX electronics aims to address these issues to produce higher quality data at lower count rates.
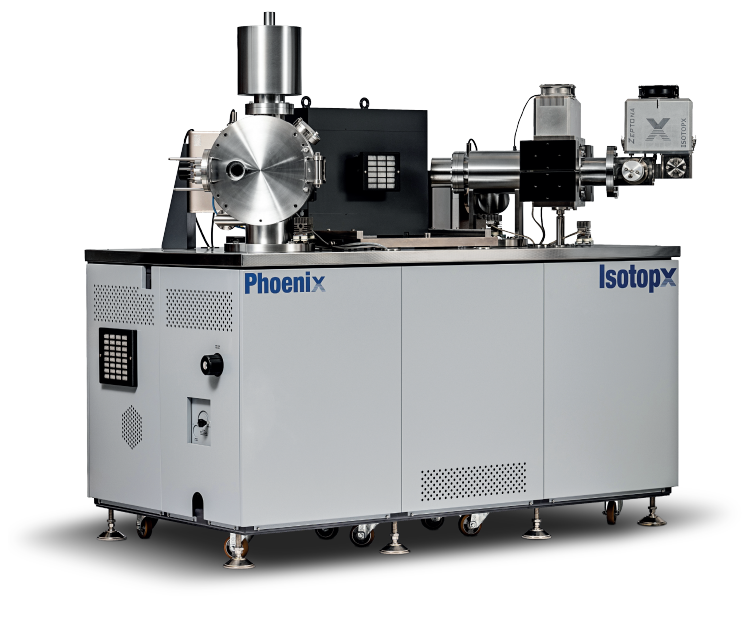
Are the peaks flat?
Peak flatness is a source of error in ion counting measurement. A slope on the flat can result in an apparent gain change depending on where on the peak flat a measurement is made. The peak flat is shown in Figure 1 below, and scans across all ion counters are shown in figure 2. The peaks are indeed flat.
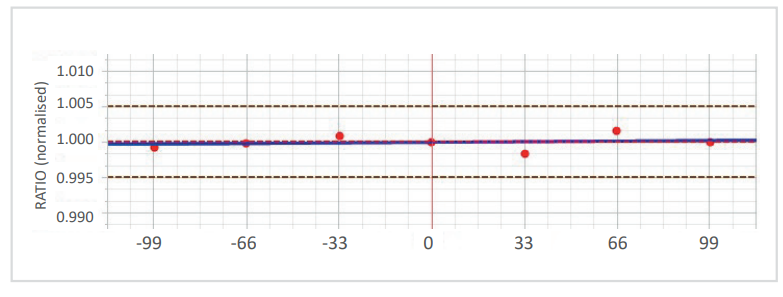
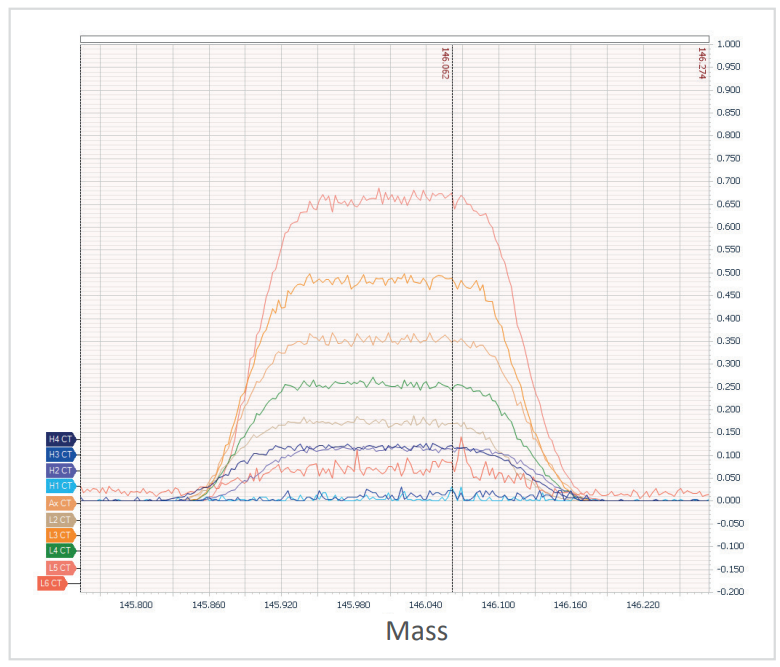
What about dark noise?
Dark noise is a measure of the counts registered on an ion counter when no beam is present. Ten channels of dark noise data were collected over several hours, shown below. In all cases the dark noise was less than 0.6 counts per minute (0.01 CPS).

Are these ion counters linear?
Ion counting detectors are inherently non-linear. This can be corrected by applying a deadtime. The stability of this deadtime and the resultant correction is critical; non-linearity will be observed as a variation of the measured isotope ratio. The linearity of the ten ion counters was determined by analysing the 234U/238U ratio of a uranium standard (NBS U500). The analysis was performed with a 238U intensity of 5e4 CPS to 3e5 CPS. Figure 3 shows typical data, demonstrating linearity to better than 0.1% 1RSD. The ion counters are linear.
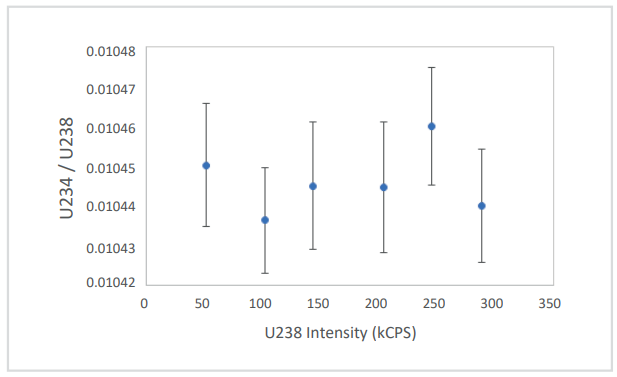
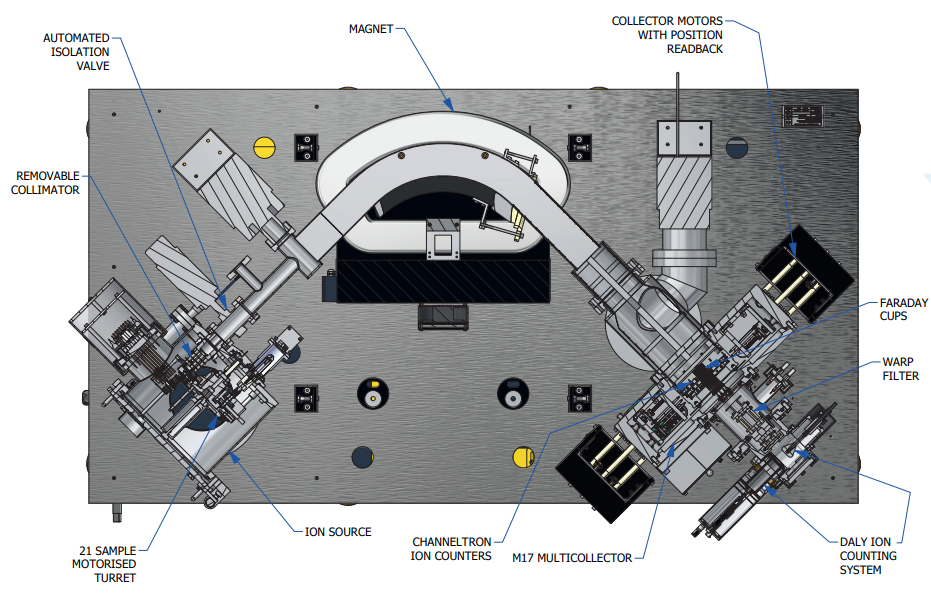
Are the gains stable?
Gain stability of ion counting detectors is another factor. The 235U/238U ratio of U500 was measured on two ion counters over 6 replicate analyse of 200 seconds at 5e4 cps 238U. The results are shown in Fig. 4, with the mean of the measured data (blue) and the certificate value (grey) indicated. No mass fractionation correction has been made, No drift was observed, the gains are stable.
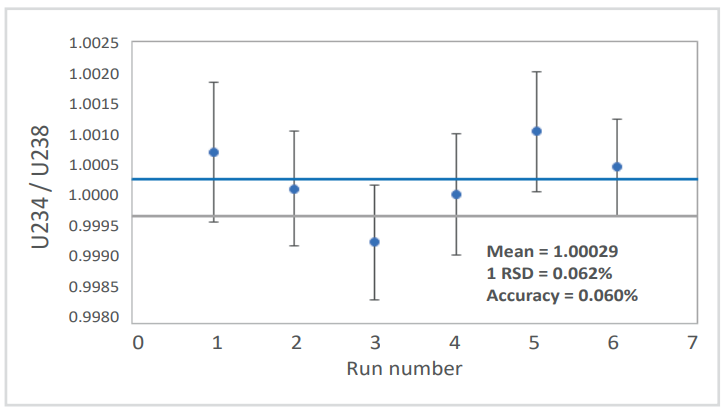
Are they stable relative to the Faradays?
Ion counter stability relative to a Zeptona Faraday was measured over 100 minutes. A 1.6e4 cps ion beam was measured for 2 s on ion counters and 10 s on the Faraday. A precision of ~0.4% 1 RSE was seen for each ion counters. An example is shown in Fig. 5, showing no evidence of ion counter gain drift versus Faradays.
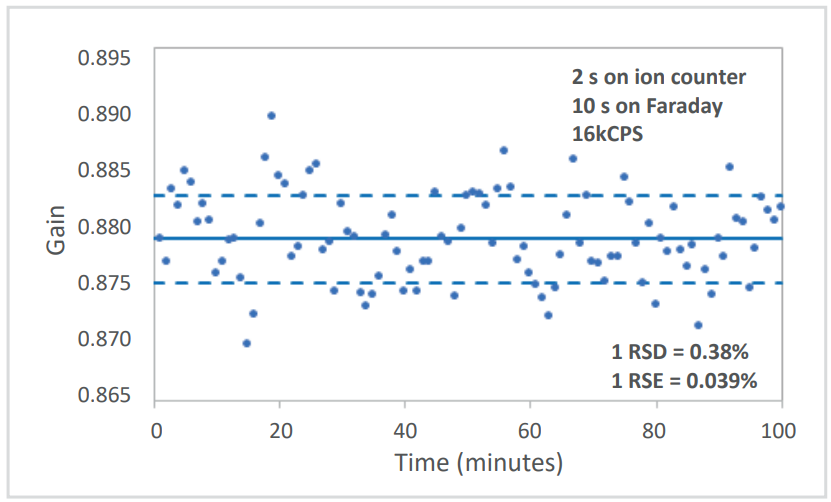
What’s a Zeptona Faraday?
The Zeptona Faraday detector employs a single channel ATONA™ amplifier and rear Faraday detector optimised for minimal noise. Figure 6 shows the noise characteristics over a range of integration times, with ATONA™ amplifier data and theoretical resistor amplifier performance shown for reference. Zeptona achieves noise levels 5 times lower than those of the ATONA™ at all integration times.
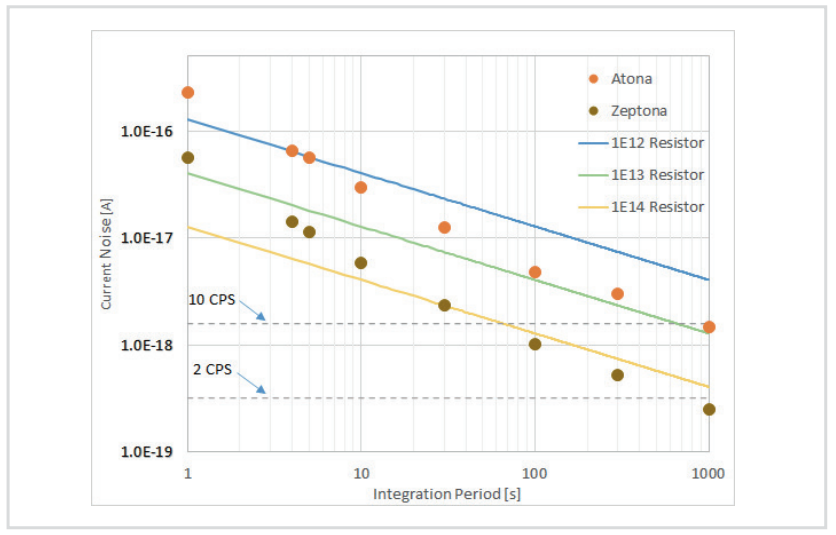
What’s the Zeptona Faraday noise level?
The Zeptona noise is comparable to the theoretical noise of a 1e14ohm resistor at integration times >10 seconds. The Zeptona Faraday was used to calibrate ion counter gain with very small ion signals. A 5e3 CPS 238U was measured on all ion counters (2 s integrations) and the Zeptona Faraday (10s integrations).
Figure 7 shows the results from a typical channel, with a precision of better than 0.15% (1RSE) achieved after 50 cycles. This demonstrates that even at very low beam intensities the Faraday noise does not limit gain calibration precision.
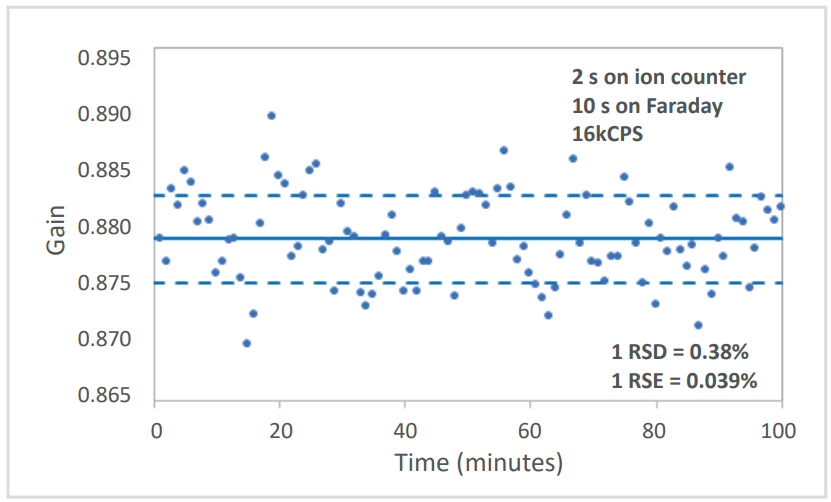
Conclusions
Our flexible, powerful multiple ion counting system offers the lowest noise and largest dynamic range:
> Up to 12 movable ion counters
> Fixed Zeptona Faraday, optional Daly detector
> Ion counting noise of 0.5 CPM, and single channel Faraday noise comparable to 1E14 Ohm resistor amplifier
> Excellent peak shape and flat over the focal plane – static and multi-dynamic ion counting analysis possible
> Stable to < 0.3% RSD over 1 hour
> Linear to < 0.3% RSD up to 300 kCPS
> Zeptona Faraday allows robust ion counter gain calibration with beams as small as a few thousand CPS
> 11 orders of magnitude dynamic range
Download Application Note
Download the complete Application Note using the link below: