I often get asked about the ATONA Faraday amplification system that we use at Isotopx. The questions are usually along the lines of “how did you come up with the idea?”, or “was it hard to develop?”, typically ending with “what’s next?”. In this blog I’ll address those questions – with the obvious limitation that the know-how behind ATONA is commercially sensitive so I won’t be divulging anything technical. I’ll also take this opportunity to get my statement in that this is a blog and therefore a personal opinion rather than a company statement. You can address any questions, comments or even disagreements directly to me (Stephen.guilfoyle@isotopx.com).
So first of all, why do it? After all, resistor-based Faraday amplification is many decades old, is extremely well understood, and is still the subject of ongoing development. To answer this I spoke to Zenon Palacz, the managing director of Isotopx and the instigator of the ATONA project. In Zenon’s words: “Simply put, we could see that the drive for ever-higher resistance amplifiers to allow the measurement at lower ion beam levels was leading to too many compromises. We knew we could do it better…”
You may already know about those compromises. For many years, isotope ratio instruments in our business, such as TIMS and MC-ICP-MS, have used Faraday-based detection for larger ion beams and/or higher abundance isotopes, and ion counting based techniques for smaller ion beams / low abundance isotopes. Fortunately, there is an overlap of dynamic range between these two detector types, meaning a cross-calibration between Faraday detector and ion counting detector is possible.
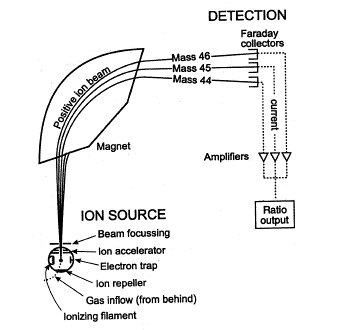
But compared to our trusty Faraday detectors, ion counting systems are less than perfect. For a start they are not perfectly linear (in other words they may respond differently to a larger ion beam than they do to a smaller ion beam). They are also less stable than Faraday detectors, sometimes exhibiting changes in efficiency over time. There are well known background noise issues too. I’m not exploring those factors today, just quoting them to illustrate the drive for more performance from Faraday detectors.
I know about all this first-hand as I worked on a three ion counter system for the VG Axiom MC-ICP-MS many years ago. I installed the first system at a highly respected lab here in the UK and the job was a huge challenge. Changing instrument tuning parameters appeared to affect the ion counter efficiencies and achieving good flat-top peak shapes proved a mammoth task. I was fortunate that the scientist that managed the lab was a very patient person, and I knew at this point that there had to be a better way.
These factors drove the development of higher-ohmic value resistor-based amplifiers. Let’s look a bit more closely. Instruments in our business most typically used 10e11 ohm amplifiers for Faraday detectors. These work very well for bigger ion beams but below about a millivolt (depending on who you talk to and what precision is required) they are less precise, meaning that ion counting systems are often used. Development scientists, both at user labs and at mass spectrometer vendors, figured that the use of higher value resistors could surmount some of these issues and enable precise Faraday analysis of smaller ion beams. This work progressed well, and before long there were Faraday amplifiers using ten and even one hundred times larger resistances (10e12 and 10e13 ohm resistors, respectively).
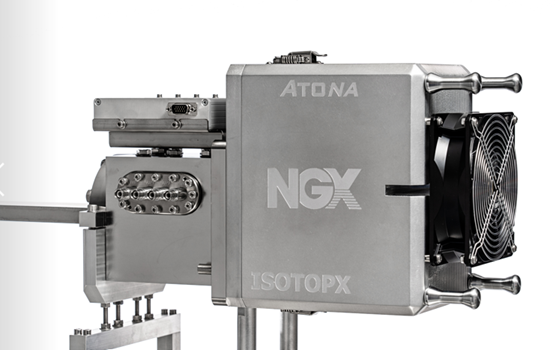
So that’s the end of that, right? Well, not exactly. Even regular users will agree that these amplifiers can be harder to use than the traditional 10e11 ohm variety. Reading the literature and consulting with users, it seems that the strength of the higher resistance value amplifiers is when you’re measuring small samples of relatively well known sample sizes and with no extreme difference in the isotopic abundance of each isotope. In these circumstances they’re ideal. But if any part of this “idealized” analysis changes they become more difficult to use.
For example, consider an instrument with 10e13 ohm amplifiers. If you have an unknown sample which happens to be larger than expected, you are either going to saturate the detector (which would maybe take many minutes or even hours to settle) or you are going to have to reduce the ion beam size to allow measurement on this detector. That’s because what you gain at the low end you lose at the high end. In other words, the 10e12 and 10e13 ohm amplifiers shift the measurable range of ion beams downwards. Deliberately reducing the ion beam size this way is not in the spirit of optimizing your precision – so your analysis is compromised.
One obvious solution is to mix the type of amplifiers used in the mass spectrometer. Again, this is excellent in theory but can be a compromise. Firstly, you have to configure your amplifier housing to suit so that specific amplifiers are attached to specific Faraday detectors. This may require an engineering visit. Then you must calibrate the gains; this is a trivial task for 10e11 ohm amplifiers but for 10e13 ohm amplifiers you cannot use a reference voltage larger than 30mv, resulting in a long calibration with poor precision. And finally, the high ohmic resistors often suffer from “resistor lag” (also known as Tau decay) – whereby the amplifier takes longer than desired to fully recover from an incident ion beam. This can mean increased analysis times.
Some of these issues can be artificially addressed using software corrections, but it is still less than an ideal solution and has been the subject of much development and optimization work in recent years. So that’s where ATONA comes in! The drive behind ATONA was to find a way to allow measurement of smaller ion beams on Faraday detectors without the compromises of higher ohmic value systems. Capacitive systems (or more precisely, capacitive transimpedance systems) have been around for a long time, but have proved to be challenging to integrate into Faraday detectors for mass spectrometers.
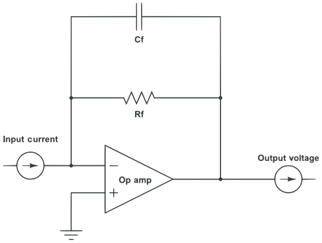
The electronics development team at Isotopx labored for quite some time to develop a solution. Were there many challenges? Of course! The choice of electronic components was critical, involving bespoke components as well as off-the-shelf varieties. The software and firmware to make this work also took time. But Zenon kept his faith in the team and the results speak for themselves.
ATONA technology has been around for a few years now, so we’ve collated quite a bit of feedback from our users. How do they find it? Is it difficult to use? What does the data look like? First of all, the overarching feedback we get is that they’re very easy to use. Gain calibrations are much less frequently required, they’re very stable, and because of the huge dynamic range compared to resistor-based Faradays, users don’t need to be as careful about larger ion beams. As with all Faraday detectors, the user can select the integration time to suit their analysis. We’ve produced a handy guide (link) to help choose the most suitable integration time depending on approximate beam intensity. So day-to-day use is easy, what about the data?
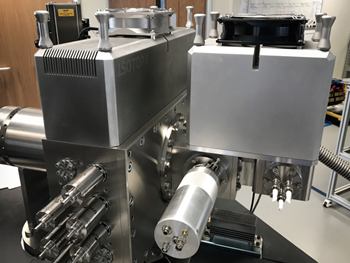
Quite a few users have now published data taken from their ATONA-equipped systems. I’ve already mentioned data published by Szymanowski et al (link) using their Phoenix TIMS, my blog article about their recent paper can give you a high-level view of what can be achieved. However, you should check out their actual paper for the full lowdown (link here, though you may need to pay for access). Similarly, the paper by Cox et al (link here) gives an excellent overview of what can be achieved with ATONA used on a noble gas mass spectrometer (the NGX).
What about the future? You may be aware that we’ve also produced a system called “Zeptona”, this takes the capabilities of ATONA and pushes them up a notch. Zeptona is typically used as a rear detector mounted behind the main detector block, optionally with an electrostatic filter to improve abundance sensitivity. It works really well with multiple ion counting systems (you can read an article about a multiple ion counting system with Zeptona here), the incredible sensitivity of Zeptona means it can be used to calibrate the ion counting detectors with less than 10,000 cps. This is a big help when you want high precision data with small ion beams.
And what’s beyond Zeptona? We never stop developing – we know our competitors are developing their own detector technology so we will do our best to stay one step ahead. Fortunately, the winners in a battle for the smartest technology are you, the users! Keep watching this blog for news of new developments…
If you have a comment about this post, or wish to point out an error or addendum, please let me know (Stephen.guilfoyle@isotopx.com).